Perhaps as many as 90 percent of all optical microscopy investigations are conducted utilizing standard achromat or plan achromat objectives, which are the cheapest, most readily available, and already installed on a large base of microscopes around the world. A majority of microscope manufacturers also offer a wide variety of objectives having unique configurations designed to perform specific functions not normally found on common laboratory microscopes.
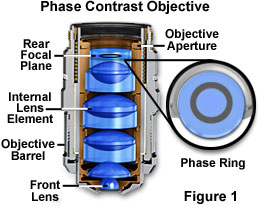
Standard brightfield objectives, corrected for varying degrees of optical aberration, are the most common and are useful for examining specimens with traditional illumination techniques such as brightfield, darkfield, oblique, and Rheinberg. Several of these methods involve modifications to the substage condenser, but still utilize standard achromat, fluorite, and/or apochromat objectives either with or without flat-field correction. Other, more complex, techniques require specific objective configurations, which often include placement of a detector on or near the rear focal plane. To complicate the issue, the objective rear focal plane usually resides in the center of an internal glass lens element, an area that is not easily accessible to the microscopist.
Objectives designed for phase contrast, Hoffman modulation contrast, and differential interference contrast require the assistance of optical detectors to modify events occurring at the objective rear focal plane. These objectives must be specially constructed to physically place the detecting element at the appropriate focal plane within the objective body. Phase contrast objectives (as discussed below) require the insertion of a phase plate containing neutral density material and/or optical wave retarders at the rear focal plane. In addition, the phase plate must be placed conjugate to a matched annular ring positioned in the substage condenser. Hoffman objectives also require a modulation plate in the objective rear focal plane that is conjugate to a slit plate in the condenser. Differential interference contrast objectives generally do not require modification (other than the utilization of strain-free optical elements), but do rely on the action of Wollaston or Nomarski prisms strategically placed to influence optical path differences between sheared light beams at the rear focal plane. Other specialized objectives rely on modified optical elements, insertion of mirrors or oblique reflectors, adjustable apertures, and/or movable elements to perform unique functions. Details concerning the specific requirements of such objectives are discussed in the remainder of this section.
Phase Contrast - This is a classical method of introducing contrast into semi-transparent, unstained specimens such as microorganisms and cells in living tissue culture, which has been employed by biologists for the past 50 years. Phase contrast manipulates phase relationships between individual light rays as they are emitted from the specimen and translates them into amplitude or brightness changes that are visible to the microscopist. A special objective is required that is fitted with a darkened circular ring or groove (phase plate) fitted into the glass near the rear focal plane of the objective as illustrated in Figure 1. In addition, the condenser must also be modified with special annular openings suited to a particular magnification and objective. Phase contrast objectives are segregated into a number of categories depending upon the construction and neutral density of internal phase rings:
- DL (Dark-Light) - DL objectives produce a dark image outline on a light gray background. These objectives are designed to furnish the strongest dark contrast in specimens having major differences in refractive indices. The DL phase contrast objective is the most popular style for examination of cells and other semi-transparent living material and is especially suited for photomicrography and digital imaging.
- DLL (Dark Lower Contrast) - Similar to the DL objective, the DLL series allows better images in brightfield and is often used as a "universal" objective in microscope systems that utilize multiple illumination modes such as fluorescence, DIC, brightfield, and darkfield.
- ADL (Apodized Dark-Light) - Recently introduced by Nikon, the apodized phase contrast ADL objectives contain a secondary neutral density ring on either side of the phase ring. Addition of the secondary rings assists in reducing unwanted "halo" effects often associated with imaging in phase contrast microscopy.
- DM (Dark-Medium) - DM objectives produce a dark image outline on a medium gray background. These objectives are designed to be used for high image contrast with specimens having small phase differences, such as fine fibers, granules, and particles.
- BM (Bright-Medium) - Often referred to as negative phase contrast, BM objectives produce a bright image outline on a medium gray background. BM objectives are ideal for visual examination of bacterial flagella, fibrin bundles, minute globules, and blood cell counting.
In order to allow the microscopist to quickly identify phase contrast objectives, many manufacturers inscribe important specifications, such as the magnification, numerical aperture, tube length correction, etc., on the outer barrel in green letters. This serves to differentiate phase contrast objectives from ordinary brightfield, polarized, DIC, and fluorescence objectives which either use an alternative color code or the standard black lettering.
Differential Interference Contrast (DIC) - Nomarski differential interference contrast is also useful for unstained specimens, but is less effective with birefringent samples and can be used with reflected light for metallography and wafer inspection. DIC objectives are not modified internally, but are designed for use with special magnification-dependent modified Wollaston or Nomarski prisms to produce high-contrast images. These objectives are also useful for brightfield, darkfield and other techniques when the prisms are removed from the optical path. Because DIC microscopy utilizes polarized light, strain must be minimized in objectives designed for this type of application. In the past, only strain-free achromats, planachromats, and some high-performance fluorite objectives were suitable for this task. However, recent improvements in lens design and antireflection coatings now allow utilization of apochromat objectives for DIC observation, photomicrography, and digital imaging. The barrel of objectives intended for use with a DIC prism is usually inscribed with the specific prism (low, medium, high, or 1, 2, 3, etc.) intended to be coupled with the objective.
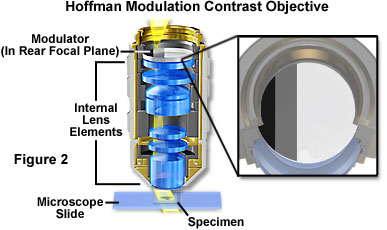
Hoffman Modulation Contrast - Objectives for the Hoffman modulation contrast system are designed to increase visibility and contrast in unstained and living material by detecting optical gradients (or slopes) and converting them into variations of light intensity. Modulation contrast objectives have a unique optical amplitude spatial filter, termed a modulator, inserted into the rear focal plane (see Figure 2) of an achromat or planachromat objective (although a higher correction factor can also be utilized). The modulator has three zones of varying neutral density that transmit either one, fifteen, or one hundred percent of the light passing through the objective. Unlike the phase plate in phase contrast objectives, Hoffman modulators are designed not to alter the phase of light passing through any of the zones. When viewed under modulation contrast optics, transparent objects that are essentially invisible in ordinary brightfield microscopy take on an apparent three-dimensional appearance dictated by phase gradients in the specimen. Recent innovations in Hoffman objective design have yielded models that allow the contrast direction to be varied using a modulator inside the objective. Once adjusted, the contrast direction is maintained over the entire magnification range in a set of matched objectives.
Infrared Microscopy - Optical microscopy in the infrared region of the electromagnetic radiation spectrum is often undertaken for the study of materials that are uniformly transparent or opaque in the visible spectrum, but have significant absorption or transmission bands in the 700 nanometer-plus wavelength region. Reflected light is the technique of choice for infrared microscopy, and several specialized catoptric objectives have been designed to capture images with infrared light reflected from opaque specimens.
Although all microscope objectives transmit some degree of the shorter infrared wavelengths, very few are corrected for aberrations in this region and show a marked shift in focus when transcending from visible to infrared light. Most manufacturers offer specialized objectives with reduced numerical apertures that are designed to increase the depth of focus in specimens imaged with infrared light. Oil immersion objectives will not function normally with standard oils and the only suitable immersion fluid that is currently available for infrared microscopy is paraffin oil. The major concern with this type of microscopy is the ability to capture satisfactory photomicrographs using conventional imaging technology. Currently, several film emulsions are available that respond to the infrared spectrum, but electronic detectors are rapidly becoming the imaging device of choice for studies in this wavelength range.
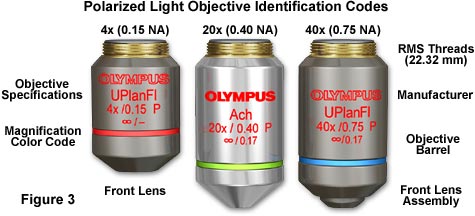
Interference Microscopy - Interferometry is applied to the study of microscopic specimens by taking advantage of interference produced when light passing through an object is caused to interfere with a reference beam of light that has followed a somewhat different pathway. Under these circumstances, the opaque surface of a reflected light specimen or transparent specimens are imaged when the path difference between the two light beams is converted into intensity fluctuations. A wide variety of microscope and objective designs have been implemented for interference microscopy, many following the basic principles of the Michelson, Mach-Zehnder, and Jamin interferometers. Manufacturers of industrial microscopes (both reflected and transmitted illumination) often produce specialized objective/microscope combinations that take advantage of the optical interference phenomenon to achieve high-precision measurements.
Polarized Light - Unlike most other forms of microscopy, polarized light microscopy produces the best images when a minimum of optical elements are used in the construction of objectives. It is important to ensure that lens elements, optical cements, and antireflection coatings are free of both strain and birefringent materials that might interfere with quantitative assessment of specimen birefringence. Apochromatic objectives, the choice for most forms of microscopy, are generally not utilized for polarized light investigations due to the high number of internal lens elements that often contribute to internal reflections and strain. Most manufacturers produce objectives that are specifically optimized for polarized light and differential interference contrast, with fluorite-class objectives being used most often for these purposes. Objectives optimized for polarized light often have the exterior portion of the barrel painted black, with the specifications inscribed in bright red letters (Figure 3).

Ultraviolet Fluor Objectives - Epi-fluorescence applications require high numerical aperture objectives in order to capture the maximum amount of light from faintly emitting fluorescent specimens. The ratio of specific specimen fluorescence to the intrinsic background fluorescence becomes a primary concern when imaging single molecule and other low-light fluorescence events. In these situations, auto-fluorescence and/or internal reflection inside the objectives can interfere with imaging small structures and low fluorescence targets.
Fluorescence objectives are designed with quartz and special glasses that have high transmission from the ultraviolet (down to 340 nanometers) through the infrared regions of the electromagnetic radiation spectrum. These objectives are extremely low in auto-fluorescence in order to optimize the luminous flux emitted as secondary fluorescence by fluorophores attached to specimens. In addition to special lens elements, ultraviolet (UV) fluor objectives utilize specialized optical cements and antireflection coatings that are designed to operate through an extended range of fluorescence excitations throughout the spectrum. Correction for optical aberration and numerical aperture values in UV fluor objectives usually approaches that of apochromats, which contributes to image brightness and enhanced resolution of images generated by these advanced lenses (Figure 4). In addition, these objectives are designed with non-fluorescent glass to minimize artifacts arising from auto-fluorescence by internal lens elements. The primary drawback of high-performance fluorescence objectives is that many are not corrected for field curvature, resulting in images that do not have uniform focus throughout the view field. Although this problem is only of secondary concern when imaging weakly fluorescent specimens (especially with laser scanning confocal microscopy), it becomes a major issue when the objective is utilized to perform under conventional illumination techniques such as brightfield, darkfield, and differential interference contrast.

Reflected Light Objectives - Transmitted light objectives designed to be used with a cover glass are unsuitable for examining reflected light specimens whose surfaces are uncovered. Instead, specialized objectives that are corrected for observation and imaging of specimens without a cover glass are employed for reflected light microscopy. Today, most reflected light microscope objectives are infinity-corrected and are available in a broad spectrum of magnifications ranging from 5x to 200x. These objectives are manufactured in various qualities of chromatic and spherical correction, ranging from simple achromats to planachromats and planapochromats. Most, but not all, are designed to be used "dry" with air in the space between the objective and specimen. Many reflected light objectives are designed to focus at a longer working distance from the specimen than is usual (see below). Such objectives are labeled on the barrel of the objective as LWD (Long Working Distance), ULWD (Ultra-Long Working Distance), and ELWD (Extra-Long Working Distance).
Objectives designed to be used with reflected darkfield illumination have a special construction consisting of a 360-degree hollow chamber surrounding the centrally located lens elements (Figure 5). Light from the illuminator passes through the periphery of the objective and is directed at the specimen from every azimuth in oblique rays to form a hollow cone of illumination. This is often accomplished by means of circular mirrors or prisms located at the bottom of the objective's hollow chamber. In this manner, the objective serves as two separate optical systems coupled coaxially such that the outer system functions as a "condenser" and the inner system as a typical objective.

The necessity of a hollow collar surrounding the lens elements in reflected light objectives requires that the diameter of the objective be significantly greater than that of ordinary brightfield objectives. In most cases, a nosepiece-mounting thread diameter larger than the Royal Microscopical Society (RMS) standard is used in reflected light objectives. This requires that reflected light darkfield objectives have a nosepiece with a larger thread size, which is typically referred to as a BD or BF/DF thread size. Most manufacturers offer objective adapters that convert standard RMS thread size nosepieces to BD thread size, allowing the use of these objectives on reflected light microscopes. Care should be taken to ensure that objectives used on BD thread nosepieces will conform to the tube length of the microscope.
Variable Numerical Aperture Objectives - Specimens with unusually high fluorescence quantum yields and/or very bright darkfield specimens often induce image flare by light emitted from areas outside the focal plane. To compensate for this artifact, manufacturers offer high numerical aperture objectives that are equipped with an internal iris diaphragm to increase image contrast during photomicrography or digital imaging. Opening or closing the iris diaphragm determines the size of the objective rear aperture yielding a variable numerical aperture range between 0.5 and the objective's upper limit (up to 1.35-1.4 with apochromatic objectives; Figure 6). Although iris diaphragms were once utilized in a wide variety of objective designs, modern variable numerical aperture objectives are usually at the high end (60x to 150x) of the magnification range.
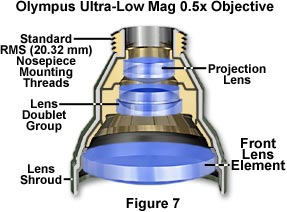
Ultra-Low Magnification Objectives - Objectives having a magnification factor below 4x are considered to be very low in magnification (see Figure 7) and may not be compatible with all microscope optical systems. Generally, Köhler illumination is difficult to achieve with low magnification objectives, which often require specialized matching condensers to fill the rear aperture with sufficient illumination. Magnifications down to 0.5x have recently been achieved, but these objectives require special tube lenses and condensers, making them useful only on the microscopes for which they were designed.
Long Working Distance (LWD) - These objectives are designed to increase the working distance over that of conventional objectives through the use of special optical elements. The most useful application for LWD objectives is to view living cells in tissue culture through the walls of the thick vessels used to support the cells. Other uses for these objectives are to image samples through thick glass plates (for instance, between two microscope slides), or when micromanipulation of specimens must be conducted while viewing. Reflected light objectives are also produced with long working distances for examination of large specimens that would ordinarily be too bulky to fit within the confines of a normal microscope optical system.