Electrophysiology is the study of the electrical properties and activity within biological systems, particularly excitable cells such as neurons and muscle cells. It involves the measurement, recording, and analysis of electrical signals generated by these cells to ultimately provide insights into their function and communication.
Here we take an in-depth look at electrophysiology, including its history, future, and how it is used in experimental techniques across fields.
History of Electrophysiology
The electrophysiology field has a rich history dating back to the 18th and 19th centuries, with the earliest instances marked by significant discoveries in understanding the electrical properties of living organisms.
Arguably, one of the most groundbreaking experiments was performed by Luigi Galvani, an Italian physician, in the late 18th century. In this experiment, Galvani observed that frog muscles contracted when touched with a metal instrument while in contact with another metal object.2
He interpreted this evidence as “animal electricity,” which contributed to the overall understanding of bioelectric phenomena. Early instances of electrophysiology, such as Galvani’s experiment, laid the groundwork for modern research in the field, leading to a deeper understanding of the electrical properties of living organisms and how to research them.
As we move further into the 21st century, scientists relied heavily on field recording, as a single cell was exceedingly difficult to locate. With the addition of a microscope, scientists could locate the cell, and with advances in optical design, such as infrared differential interference contrast (IR-DIC), researchers could visualize deeper into live tissue.
When combined with a high-speed camera, this microscope setup enabled the observation of internal cellular dynamics in real-time with methods such as calcium imaging. Now solutions such as the fixed-stage electrophysiology upright microscope to more advanced imaging solutions, such as laser scanning confocal and multiphoton microscopes, are revolutionizing the field of electrophysiology for advancing research.
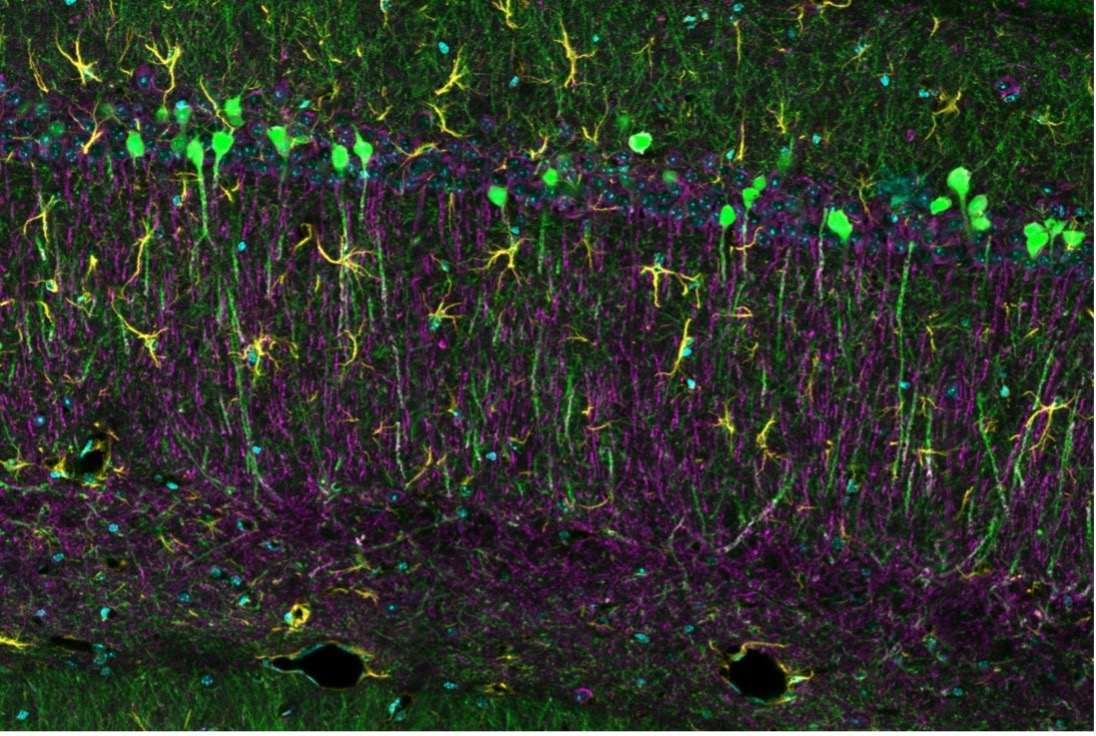
The coronal section of an H-line mouse brain, cyan; DAPI (cell nuclei), green; YFP (neuron), yellow; Cy3 astrocytes, magenta; AlexaFluor 750 (microtubule). A total of 77 four-channel XYZ positions (11 × 7) were acquired using a 1K resonant scanner within 16 minutes to create the stitched image, which used to require two hours using a galvanometer scanner. Sample courtesy of Takako Kogure and Atsushi Miyawaki, Laboratory for Cell Function Dynamics, RIKEN CBS.
What Is Electrophysiology Used for?
In today’s research landscape, electrophysiology can be found in common fields, including cardiology, neuroscience, and many more. In cardiology, the most common use of electrophysiology is the electrocardiogram, or ECG.
An ECG is an electrophysiological diagnostic device that measures and records the electrical activity of the heart. Electrodes placed on the skin detect the electrical signals generated by cardiac cells. The ECG provides valuable information about the heart’s rhythm, rate, and overall electrical function, making it an essential tool in the diagnosis and management of cardiovascular diseases.
In the context of neuroscience, electrophysiology is often used to study the electrical properties of neurons, including how they generate and transmit electrical signals.
There are two main approaches in patch-clamp electrophysiology: intracellular and extracellular. Intracellular electrophysiology involves measuring the electrical potential across the membrane of a single cell. This provides detailed information about the cell’s membrane potential and any changes that occur during cellular activities, like action potentials.
Membrane potential is an intrinsic property of all cells that results from excess negative or positive charge on either side of the cell membrane.1 An action potential is a brief but large electrical depolarization and repolarization of the neuronal plasma membrane caused by exchange of positively charged ions.1
Patch clamp recording is widely used to study the electrical activity of individual cells, particularly neurons. It involves placing a glass micropipette electrode against the cell membrane to form a tight seal, enabling the measurement of membrane potential changes with high precision. Patch clamping can be performed in whole-cell, cell-attached, inside-out, or outside-out configurations, each suitable for a different experimental purpose.
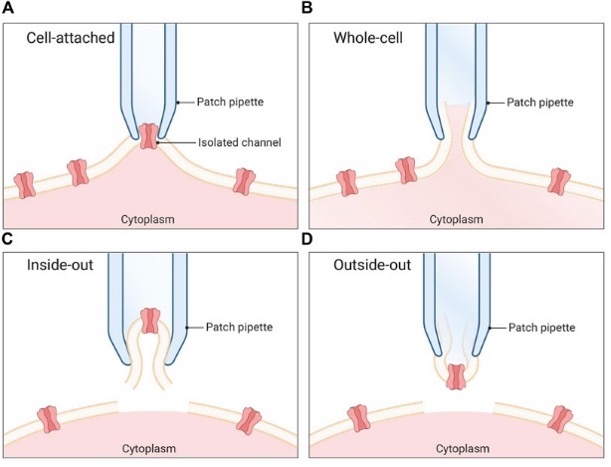
Patch clamp recording configurations. (A) Cell-attached: enables measurement of the current of a single channel. (B) Whole-cell: enables measurement of the current from an entire cell. (C) Inside-out: enables measurement of the current across a small patch whose cytosolic side faces outward. (D) Outside-out: enables measurement of the current across a small patch whose extracellular side faces outward. Image source: Ahmadi, Shirin et al. 2023 (Frontiers).3
Voltage clamp recording is a method used to measure and control the membrane potential of a cell while simultaneously measuring the ionic current flowing across the cell membrane.2 This technique lets researchers study the properties of ion channels and receptors to ultimately learn more about the mechanism that causes an action potential.
Current clamp recording involves injecting current into a cell while measuring changes in membrane potential. This technique is used to study the intrinsic electrical properties of neurons, such as resting membrane potential and action potential firing patterns.
On the other hand, extracellular electrophysiology involves measuring electrical activity from multiple cells or over a larger area. This approach is commonly used to study the overall activity and interaction of neural networks. Multi-electrode arrays, or MEAs, enable simultaneous recording from multiple sites, providing insight into network-level activity.
To summarize, the difference between intracellular and extracellular electrophysiology lies in the location of the recording electrode in relation to the cell membrane. Intracellular recordings provide information regarding the electrical properties of individual cells, while extracellular recordings capture information from the collective activity of a group of cells. Both approaches are highly valuable for understanding the function of biological systems.
Future of Electrophysiology
The future of electrophysiology holds exciting possibilities driven by the continued advancements in technology and methodology. We’re already seeing the need for advancements in data analysis and computational modeling as the complexity of electrophysiological data sets increases. Machine learning tools will likely play a pivotal role in extracting meaningful insights from large datasets. Development of miniaturized and wireless devices has already begun and will continue to enable minimally invasive monitoring of electrical activity in the body.
Optogenetics is an example of a cutting-edge technology that relies on microscopy and optics to control the activity of specific cells, typically neurons, with light. The method involves the genetic modification of neurons to introduce light-sensitive protein channels. Once expressed, shining a light on a defined region of the cell, an ion concentration can be changed locally, and the effects can be observed in real-time under a microscope.1
This level of insight and visibility into cellular activity opens opportunities for studying neural circuits, brain functions, and neurological disorders, as well as the development of therapeutic interventions.4 Overall, the future of electrophysiology holds tremendous promise for advancing our understanding of biological systems, diagnosing, and treating disease.
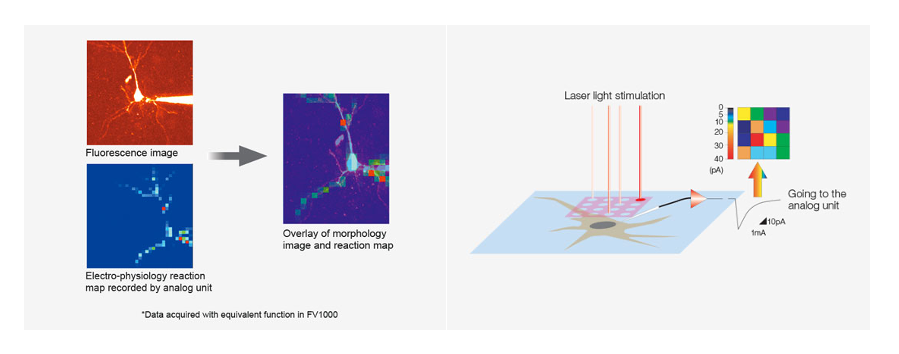
Example of a 3D stimulation reaction map for measuring electrophysiological response. To achieve highly targeted laser light stimulation, the observation field is divided into a grid, and the laser illuminates each area in a pseudorandom sequence that avoids sequential stimulation of adjacent areas. A stimulation reaction map is drawn based on the patch clamp recording or imaging intensity. Integration of an optional piezo nosepiece extends the reaction map to 3D, with stimulation delivered at depths different from the imaging plane. Image data courtesy of Haruo Kasai Center for Disease Biology and Integrative Medicine, Faculty of Medicine, the University of Tokyo. Captured on a FLUOVIEW laser scanning microscope.
Key Takeaways about Electrophysiology
In the end, electrophysiology stands at the forefront of how we understand the intricacies of living organisms, particularly in the neuroscience and cardiac spaces. By unraveling the mysteries of cellular electrical activity, researchers can unlock insights into the fundamental mechanisms behind neural communication, muscles contraction, and many more physiological processes.
From the pioneering experiments lead by early scientists, such as Galvani, to the modern, cutting-edge technologies of today, the field of electrophysiology has undergone remarkable innovation driven by collaboration and the pursuit of knowledge. Through continued exploration, we can unlock even deeper insights into the electrical activity that powers the human body.
References
- Hardin, Jeff et al. Becker’s World of the Cell, 9th Global Edition. Pearson, 2016.
- Molleman, Areles. Patch Clamping: An Introductory Guide to Patch Clamp Electrophysiology. John Wiley Sons, 2008.
- Ahmadi, Shirin et al. “From Squid Giant Axon to Automated Patch-Clamp: Electrophysiology in Venom and Antivenom Research.” Frontiers, 2023.
- Häusser, Michael. “Optogenetics - The Might of Light.” New England Journal of Medicine, 2021.
Related Content
Brochure: Fixed Stage Microscopes for Physiological Experiments
Behind the Scenes: Developing the FLUOVIEW FV4000 Confocal Microscope
Webinar: Transforming Precision Imaging with a Confocal Microscope